Dynamic Microgrids for Strengthening Power System Resilience
Written by Jose Luis Domínguez-García, Antonio Pepiciello, Pol Paradell, and Anzhelika Ivanova
Microgrids will have a crucial role in enhancing power system resilience as they can supply the load locally, through distributed energy resources, when the connection to the main grid is not available. In this article, we introduce the concept of dynamic microgrids, time-variant networks of microgrids forming the main power grid, to lower the risks of load shedding and fault propagation. Furthermore, we describe an intentional islanding procedure enabled by smart grid technologies and based on a two-step process: the identification of balanced microgrids connected to the grid and their optimal disconnection to minimize global load shedding. The expected benefits of implementing dynamic microgrids are a significant reduction of the expected loss of load, strong mitigation of the consequences of high-impact low-probability events, and stability enhancement through the limitation of fault propagation.
A microgrid can be defined as a locally controlled cluster of Distributed Energy Resources (DERs) and loads at the LV and MV levels [1]. The main aim of the coordinated control is to maintain the energy balance locally while keeping all the components of the microgrid within their technical constraints. Microgrids can be classified into isolated and grid-tied. Isolated microgrids are mainly used for the electrification of remote areas or geographical islands [2], while grid-tied microgrids are connected to the main grid. The deployment of smart grid technologies, like bidirectional inverters and advanced monitoring and control systems played a crucial role in enabling the technical feasibility of grid-tied microgrids [3]. With the help of these technologies, grid-tied microgrids can now be safely disconnected and resynchronized to the main grid and provide a wide range of services. The development of microgrids has coincided with a rise in the frequency of extreme weather events and intentional cyber-physical attacks on power grids worldwide. This led to the distinction between the concept of reliability, covered by N-1 and N-2 contingency analyses in power systems to the concept of resilience, involving the study of the so-called black swan events and the formulation of the resilience trapezoid [4].
Figure 1. Microgrid enhancement of resilience trapezoid. The orange line represents the mitigating effect of intentional islanding on the resilience level of the system during an extreme event (Readapted from [4] and [5]).
Microgrids can play a key role in each of the phases: prevention, correction, emergency coordination, restoration, and adaptation (see Figure 1). This article focuses on a particular solution to prevent complete black-out and minimize loss of load after extreme events, increasing the resilience of the system: intentional islanding.
Intentional islanding is the anticipated and systematic separation of the main grid from the power network to avoid a major breakdown. The separated dynamic microgrids correspond to islands of the main grid. The main reason for this separation is to overcome complete blackouts and cascading faults, increasing the resilience of the system. This separation is also employed for system maintenance, voltage enhancement, power-loss scenarios, temporary faults, and improving the efficiency of the network. Once the microgrid is disconnected, it must be able to maintain the energy balance locally, using the available DERs and the associated controls to keep frequency and voltage stable.
If equipped with specific monitoring, control, and reconfiguration capabilities (enabled by technologies such as multiport converters) power grids can be considered as sets of microgrids with a time-varying structure. Depending on the local energy balances and the coherency of the system, these grids may be referred to as dynamic microgrids. As opposed to the steady topology of static microgrids, dynamic microgrids change depending on the operating conditions of the power system. Their disconnection can be optimally planned in order to minimize the impact of contingencies and faults on the network. A graphical example of the islanding process is shown in Figure 2.
Figure 2. Graphical visualization of dynamic microgrids resulting from intentional islanding.
A flow chart describing the process for determining the structure of the dynamic microgrids and their optimal disconnection is shown in Figure 3. The process involves the following steps:
- Detection/Forecast of Propagating Fault: The grid works or is expected to work under disturbed conditions.
- Intentional Islanding:
- Islands Check:
- Energy balance - Check if the microgrid can maintain energy balance, i.e. if the generation in the island can supply the demand.
- Coherency evaluation - Check the synchronization of generators in the island to ensure island stability.
- Islands Detection:
- Clustering - Determination of the islands with different solution techniques including Mixed Integer Linear and Nonlinear Programming, meta-heuristic methods, [6] and machine learning [7].
- Control capabilities – Check the capabilities of the islands to control the voltage and frequency.
- Islands Disconnection from the Main Grid: The islands are disconnected from the main grid to stop the propagation of the fault and maintain the power supply in all parts of the grid.
- Islands Check:
- Reconnection to the main grid: after the fault is cleared or the threat is over, islands are resynchronized to the grid.
Figure 3. raphical representation of the intentional islanding process.
The described approach aims to improve the resilience level of the power grid, although it requires a homogeneous presence of DERs, grid-forming devices, a high degree of reconfiguration, and advanced monitoring systems.
Conclusion
Distributed, reconfigurable and intelligent decisions may lead to an optimized, resilient, secure, and stable operation; in this line, Dynamic Microgrids may become enablers and integrators of new solutions for ensuring the continuity of service for the consumers; taking advantage of the flexibility and potential reconfiguration options on the distribution grid with several active agents.
References
- N. Hatziargyriou, H. Asano, R. Iravani and C. Marnay, "Microgrids," IEEE power and energy magazine, vol. 5, p. 78–94, 2007.
- X. Lu and J. Wang, "A game changer: Electrifying remote communities by using isolated microgrids," IEEE Electrification Magazine, vol. 5, p. 56–63, 2017.
- P. Asmus, "Why Microgrids Are Moving into the Mainstream: Improving the efficiency of the larger power grid.," IEEE Electrification Magazine, vol. 2, p. 12–19, 2014.
- R. Moreno, M. Panteli, P. Mancarella, H. Rudnick, T. Lagos, A. Navarro, F. Ordonez and J. C. Araneda, "From reliability to resilience: Planning the grid against the extremes," IEEE Power and Energy Magazine, vol. 18, p. 41–53, 2020.
- M. Panteli and P. Mancarella, "The grid: Stronger, bigger, smarter?: Presenting a conceptual framework of power system resilience," IEEE Power and Energy Magazine, vol. 13, p. 58–66, 2015.
- A. Ivanova, P. Paradell, J. L. Domı́nguez-Garcı́a and A. Colet, "Intentional Islanding of Electricity Grids Using Binary Genetic Algorithm," in 2020 2nd Global Power, Energy and Communication Conference (GPECOM), 2020.
- F. D. Caro, A. Pepiciello, F. Milano and A. Vaccaro, "Measurement-based coherency detection through Monte Carlo Consensus Clustering," Electric Power Systems Research, vol. 216, p. 109075, 2023.
This article was edited by Cesar Duarte.
To view all articles in this issue, please go to April 2023 eNewsletter. For a downloadable copy, please visit the IEEE Smart Cities Resource Center.
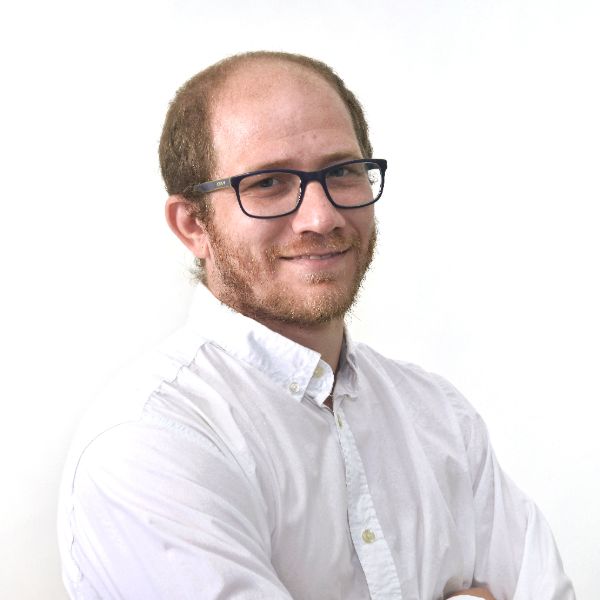
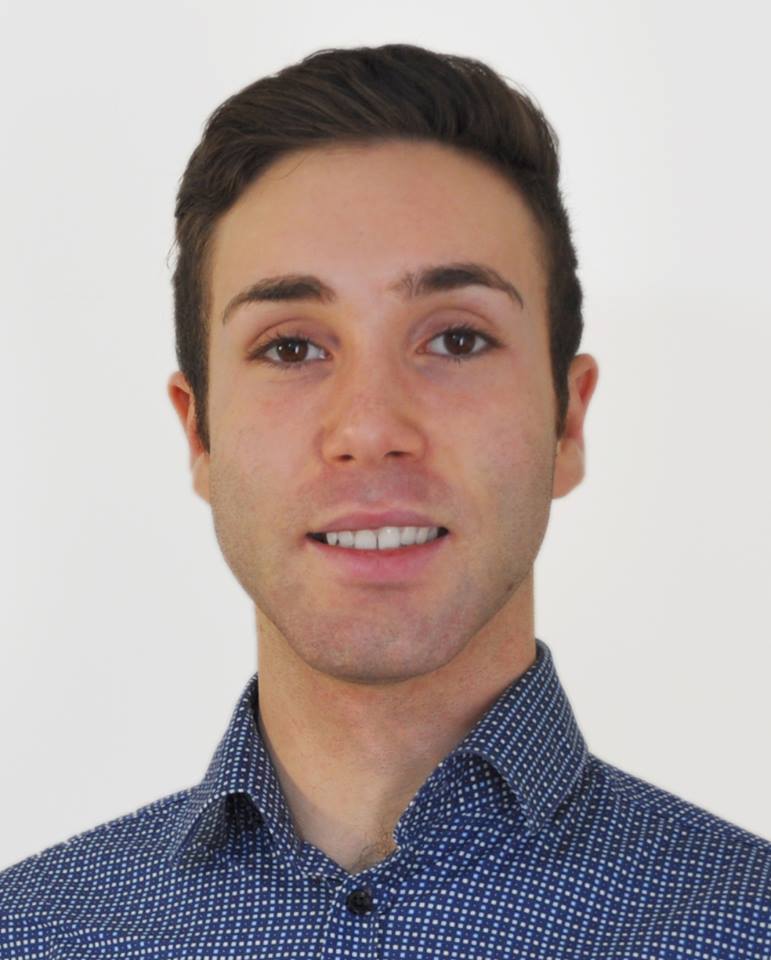
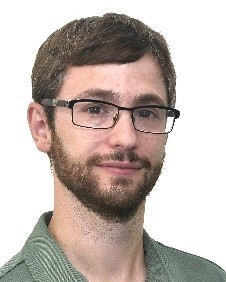
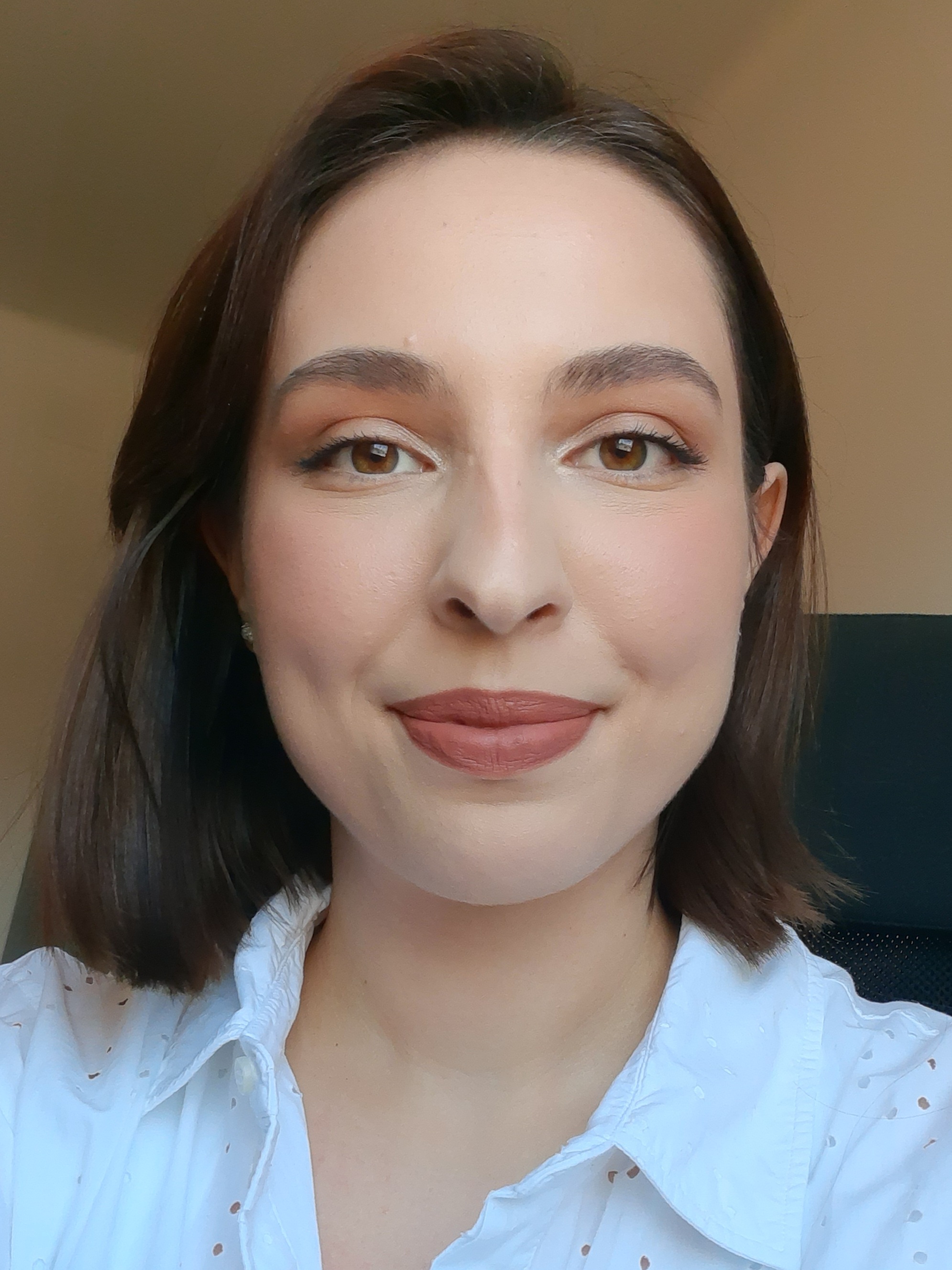
To have the Bulletin delivered monthly to your inbox, join the IEEE Smart Grid Community.
Past Issues
To view archived articles, and issues, which deliver rich insight into the forces shaping the future of the smart grid. Older Bulletins (formerly eNewsletter) can be found here. To download full issues, visit the publications section of the IEEE Smart Grid Resource Center.