Smart Grid and Zero-Emissions Energy Systems: The Need for a Multi-Dimensional Investment Planning Perspective
By Soheil Mohseni and Alan Brent
Nearly 200 countries met in Paris in December 2015 and agreed on aligning their energy portfolios to the global greenhouse gas emission reduction goals. However, without using cutting-edge technology, the countries may not reach the sustainable development goals set by the Paris Agreement. Although there is no penalty for countries that fall short of the promised target, this will definitely have a negative consequence on the collective efforts to address the climate change challenge. Smart grid technologies will offer ways to integrate the renewable energy sources and to promote demand-side management programs. However, due to the high level of risk associated with green energy investments, it is essential to consider several factors in the planning phase of the sustainable energy systems in the smart grid milieu.
As a relatively new player in the context of smart grids, a sustainable energy system can pave the way for a cost-effective solution. Optimal investment planning for sustainable energy systems are usually obtained by optimizing an objective function considering the constraints related to the operation of the components for a sample year. In this way, mathematical models of the components, in addition to the long-term energy consumption and meteorological forecasts, are used in a computer program as the input data, and the optimal sizing for the system components are obtained as the decision variables. In this regard, a proper investment planning modeling framework for a sustainable energy system should integrate the following considerations to reflect the real-world practice:
1. Uncertain parameters: Modeling the uncertainties associated with the various input parameters of the system.
2. Demand response program: Enabling the active customers to participate in the energy management of the system.
3. Policy intervention: Implementation of a policy intervention in support of private sector participation in renewable energy investment.
Uncertain Parameters:
As stated earlier, energy consumption and meteorological data (e.g. wind speed and solar irradiance), are considered as the main input data in an investment planning modeling framework for a sustainable energy system, including weather-driven sources. Considering uncertainties associated with input data (e.g. due to the stochastic nature of weather), in the optimal investment planning procedure of a sustainable energy system results in a more realistic system reliability analysis and total cost estimation.
In fact, if system uncertainties are not taken into account, the actual cost-effective and feasible combination of system components sizing may be different from the calculated optimal solution. Therefore, it is necessary to model the uncertainties associated with the forecasted parameters of a sustainable energy system, while implementing an optimal investment planning strategy.
A proper treatment of the uncertain parameters of the sustainable energy systems plays a key role in taking appropriate and cost-effective decisions regarding the optimal size of the components by investment planners. The uncertainties associated with the availability of the weather-driven sources, such as wind and solar, as well as the uncertainty associated with energy demand forecast, have the most significant impacts on the optimal sizing of the components. However, it is evident that considering other sources of uncertainty, such as energy prices or load growth, in addition to those mentioned above, will make the simulation results more accurate and closer to the real-world scenarios.
Demand Response Program:
Conventional power network planning does not take load management scheme into account. However, the advent of smart grid technologies propelled the integration of demand response programs into the sustainable energy systems by providing the required information and communication technology infrastructures.
Considering the potential benefits of demand response programs, while optimally designing a sustainable energy system, can significantly reduce the optimal size of the components, which in turn reduces the overall cost of the system. The reduction of the size of components may occur due to three reasons: (i) the need for lower capacities of power generation units during the time periods that weather-driven sources are available, (ii) the need for lower capacities of energy storage units during the time periods that weather-driven sources are not available, and (iii) in the case of non-autonomous sustainable energy systems, the need for buying less electricity from the upstream power network, which is often generated by fossil-based electricity generation plants.
Policy Intervention:
In order to ensure the financial sustainability of sustainable energy systems, especially in the case of rural and island communities, electricity prices must allow cost recuperation and, therefore, policy interventions may be essential to promote affordability to the end-users. These approaches must adjust the need to secure and pull in speculation and the commitment to advance social and financial improvement among a nation, especially remote areas. In this regard, several strategies, such as subsidies, tax incentives, and loan guarantees, have been advanced to build private segment support in sustainable energy programs. The UK’s leadership in renewable energy, with almost 30 percent of the country’s electricity in 2017 generated by clean energy sources, is a good example of the effectiveness of devising appropriate policy intervention frameworks to promote renewable energy. The introduction of Climate Change Act in 2008, which is the basis for the UK’s approach to tackling climate change, has positioned the UK as a leader in green finance. The activities and ventures that will be expected to meet the Paris commitments will guarantee the move to clean development will be at the bleeding edge of policy and financial decisions made by governments and organizations in the coming decades. Hence, in line with national and regional energy planning strategies, it is essential to consider such policy interventions while optimally planning sustainable energy systems.
In summary, investment decisions and long-term planning of sustainable energy systems must necessarily cope with the uncertainties and consider the appropriate flexible load schemes and policy interventions with the goal of reducing greenhouse gas emissions under the smart grid paradigm to realize the sustainable development goals.
This article was edited by Pardis Khayyer.
Contributors
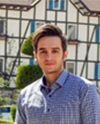
Soheil Mohseni focuses his research on developing a self-sustainable energy system for island communities using meta-heuristic optimization algorithms. His research interests include power system operation and planning, smart energy hubs, smart grids, and sustainable energy systems. Soheilholds a BSc in electrical power engineering from Kermanshah University of Technology, Iran. He also holds an MSc with high distinction in electrical power engineering, and power systems from University of Guilan, Iran. He is currently pursuing a PhD at Victoria University of Wellington, in the Sustainable Energy Systems group.
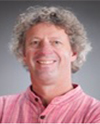
Alan Brent is inaugural Chair of Sustainable Energy Systems at Victoria University of Wellington. His research revolves around sustainable technology management, with an emphasis on the energy sector. Before joining Victoria University, he was a professor of engineering management and sustainable systems in the Department of Industrial Engineering, and the associate director of the Centre for Renewable and Sustainable Energy Studies, at Stellenbosch University in South Africa. In 2017, he was appointed as an extraordinary professor in the Department of Industrial Engineering at Stellenbosch University. In 2017, he was also appointed as a part-time professor of sustainable life cycle management in the Graduate School of Technology Management, at the University of Pretoria in South Africa. He holds bachelor degrees in engineering (chemical) and philosophy (sustainable development); master degrees in science (environmental engineering), engineering (technology management), and philosophy (sustainable development); and a PhD in engineering management.
To have the Bulletin delivered monthly to your inbox, join the IEEE Smart Grid Community.
Past Issues
To view archived articles, and issues, which deliver rich insight into the forces shaping the future of the smart grid. Older Bulletins (formerly eNewsletter) can be found here. To download full issues, visit the publications section of the IEEE Smart Grid Resource Center.